Professor Robin Bell
“Warming, caused by humans, has triggered rapid change in Antarctica’s ice and rising sea levels around the globe. Improved understanding of ice change through international collaborations must be linked with innovative strategies for coastal communities. Increasing the diversity of science is essential for an equitable sustainable thriving future for all.”
Professor Robin Elizabeth Bell, 2023 Fulbright Distinguished Chair in Science, Technology and Innovation, Funded by CSIRO
Columbia University to CSIRO | Climate Change
Robin, a Lamont Research Professor at Columbia University, studies how Antarctic ice is changing, and works with coastal communities developing scientifically informed responses to changing sea levels. She has led seven major Antarctic expeditions discovering an active volcano, large lakes and backwards-flowing rivers in a hidden mountain range beneath the ice.-
Through her Fulbright Distinguished Chair Scholarship at CSIRO, Robin will advance an international plan to study ice sheet change and investigate improved support for coastal communities undergoing rapid environmental change. A champion of women in science, she will use evidence-based approaches to advance the diversity of the academic and research workforces.
Find out more about the current cohort of Fulbright Scholars HERE.
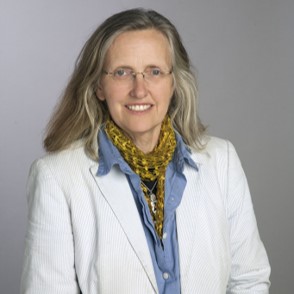
Copyright © 2021 – Fulbright